How Planes Fly

NASA has a very well written and illustrated web page that presents the standard explanation of how it is that an airplane (or rotorcraft such a helicopter or small drone) can fly. You can find it here. I've walked with a lot of people through a Boeing factory and invariably, someone in the group will express amazement that something so big can get off the ground.
I have then taken people through the standard explanation many times, and for many if not most, it just doesn't get them over the hump of understanding. Something is missing. I finally figured out how to get them over this challenge, and I'll share it here.
The key is to start by talking about a reality that our bodies are evolved to tune out. That is the pressure of the air that is on us all the time.
I'm going to express a little bit of disappointment in the way chemists have developed their fundamental gas laws. There is one key observation that they just take for granted instead of taking the time to explicitly state and provide with a name. Physicists do step in partially with the discussions that lead to the concept of entropy with this little ditty:
Whenever an imbalance in pressure occurs, substances tend to flow to restore an equilibrium.
That's nice, but it's not quite on point for what we need here. It's close. The words we need are these:
Gases exert a uniform pressure in all directions unless disturbed.
Really, that should be a law named for some old codger from the 18th century, but alas, that is not the case. Everyone just assumes that it's obvious. So hopefully we can start there. Hold out your hand. Do you feel and differences in the pressure of the air anyplace on the surface of your extended hand? Of course not. You probably don't feel much of anything at all. But, the reality is that the surface area of the human hand for adults, including your fingers, ranges from about 27 square inches (~172 cm2) for someone with an extra small glove size, up to around 60 square inches (~387 cm2) for someone who requires an XXL sized one. At seal level, where the air pressure is 14.7 pounds per square inch, that means that even someone with very small hands is dealing with almost 400 pounds of pressure on their each of their hands. For that person with the big XXL glove size, it's just short of 900 pounds! That's a lot of pressure.
Let's go a bit further. Now we'll switch to averages. The figure that anatomists use for the average surface area of an adult male is 18,000cm2 and 16,000cm2 for women. At sea level those equate to just over 20 tons of air pressure pressing on the average adult male and nearly 18.5 tons for the average female. Now let that sink in a moment. How many tons of pressure are pressing on your body right now?
That's what's missing from NASA's explanation.
But then of course I have to take exception with the way they lead into things as well. Everything they say is exactly correct, but their explanation fails to make it nice and personal. So let's say that we switch from a human standing still for a moment and talk about a dog sticking his head of of the window of a moving car as they so very much like to do. A dog doing that experiences a couple of things. One, is that the pressure on their face goes up, which seems to be the part they like so much. But another interesting part of it is that because the pressure goes up on a dog's face, it must go down someplace else in order to maintain an equilibrium.

If you are holding your dog so they won't be tempted to climb out (some dogs!) you can feel the fur on the back of their head, it will be cooler until the sensation moves to the back of your hand. That's the pressure drop you are feeling. When the dog, or your hand, or an airplane's wing moves through the air, the pressure goes up on the surfaces facing into the wind (i.e. the direction of travel), and it goes down someplace else to compensate. We do have an 18th century guy names Daniel Bernoulli who did describe this effect, and it is aptly named for him. Although, the guy who developed the math that describes it in a way that is useful for designing airplanes was Leonhard Euler.
Now, let's consider the wing of a plane since we have brought it into this discussion.
A 737-800 has a total wing area of about 1,344 square feet for both wings. Now that's not the number we really want, since the wing area specification for an airplane is is not the total surface area of the aluminum structure, but rather the surface area of its shadow. This makes sense when working with a number of equations but we want to get to how many tons of air pressure are being exerted on it when it is standing still at the gate. With the flaps and leading edge retracted, just one wing is covered with roughly 1400 square feet of aluminum and a carbon fiber composite material. So at rest there is well over 120,000 tons of air pressure on it. That is one heck of a lot of air pressure. Perhaps not surprisingly, that is not a whole lot less than the maximum take-off weight of the whole plane, and remember, we have two wings, plus we also get a little help from the shape of the fuselage and the horizontal stabilizer (that small wing-like structure on the tail).
The size of a wing required to lift a given amount of weight is proportional to to that weight. At the speeds commercial airplanes fly, it is ok for the layperson to think that the factor is about 2x. That is, the amount of static air pressure on a plane's wings when parked at the gate of an airport that is not too far above sea level is going to be about double the maximum takeoff weight of the plane. So the shape of the plane has to be such that has it gains speed going down the runway, an amount of pressure equal to the weight of the plane is removed from its upper surface.
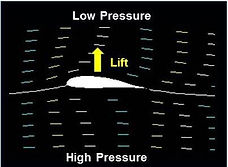
So the trick is to move the pressure around such that pressure on the top surface of the wing goes down, pressure on the lower surface stays close to the same, and it goes way way up on the leading edge.
Speed through the air moves the pressure to the leading edge. And the shape of the wing causes most of the drop to be on the top surface, which makes it colder in flight by the way.
Here is that illustration from the NASA site. I wish it had some arrows pointing at the leading edge, because that's a really big deal for making this work. On any big plane, in order for it to gain sufficient lift at a safe speed on a runway when taking off, the leading edge as presented to the wind is made dramatically larger by extending a chunk forward and down. That increases the pressure on it quite a bit, and thus subtracts an equal amount of pressure elsewhere. And here is where that bit NASA talks about the air moving faster over the top surface comes in. If it is moving, a molecule of anything, but especially a gas or a liquid, can only apply pressure in one direction at a time, which is the direction it is moving of course. So by making the wing bulge upward from the leading edge, the air rushing over it's surface is deflected up a bit. The under side of the wing remains flat in level flight, but on take-off and landing, the surface area is greatly expanded by extending flaps to the rear and down a bit. This increases the area of reduced pressure on the top of the wing.
We always call these effects on a wing (or a spinning rotor on a helicopter) lift. But, we could just as easily call it suction. Whatever, all of those thousands of tons of pressure differential makes. Now, if one thinks about all of those tons of pressure on our own bodies, and how much bigger a plane is, hopefully one can begin to get a sense that a plane, no matter how big, has no choice but to fly, unless of course one messes with Bernoulli, and we never want to do that.
Accidental lift can be a thing too. The 1959 and 1960 Chevrolets had a problem with this that caused a few accidents. They had what were in effect tiny stub wings in the back which could lift the car's rear end at high speeds.
